HapMotion
September 2019 - December 2019
Role: Principle Researcher / Developer
Arduino + Copper Wire + Magnets
Is there a way to transmit information haptically? So much of digital information is transmitted acoustically and vibrotactilely. The majority of smart watches and phones utilitize notifications with monotonous vibrations and dings - a stark departure from the rich verbal and tactile vocabulary humans possess. Perhaps there is a way to transmit information with more emotion and human-ness? This project explores information transmission through a haptic solenoidal wearable device, with individually programmable cells. Upon completion, the efficacy of this project was explored in a Psychology Department led research study, under the direction of Professor Roberta Klatzky, and Professor Lining Yao.
Preliminary Research
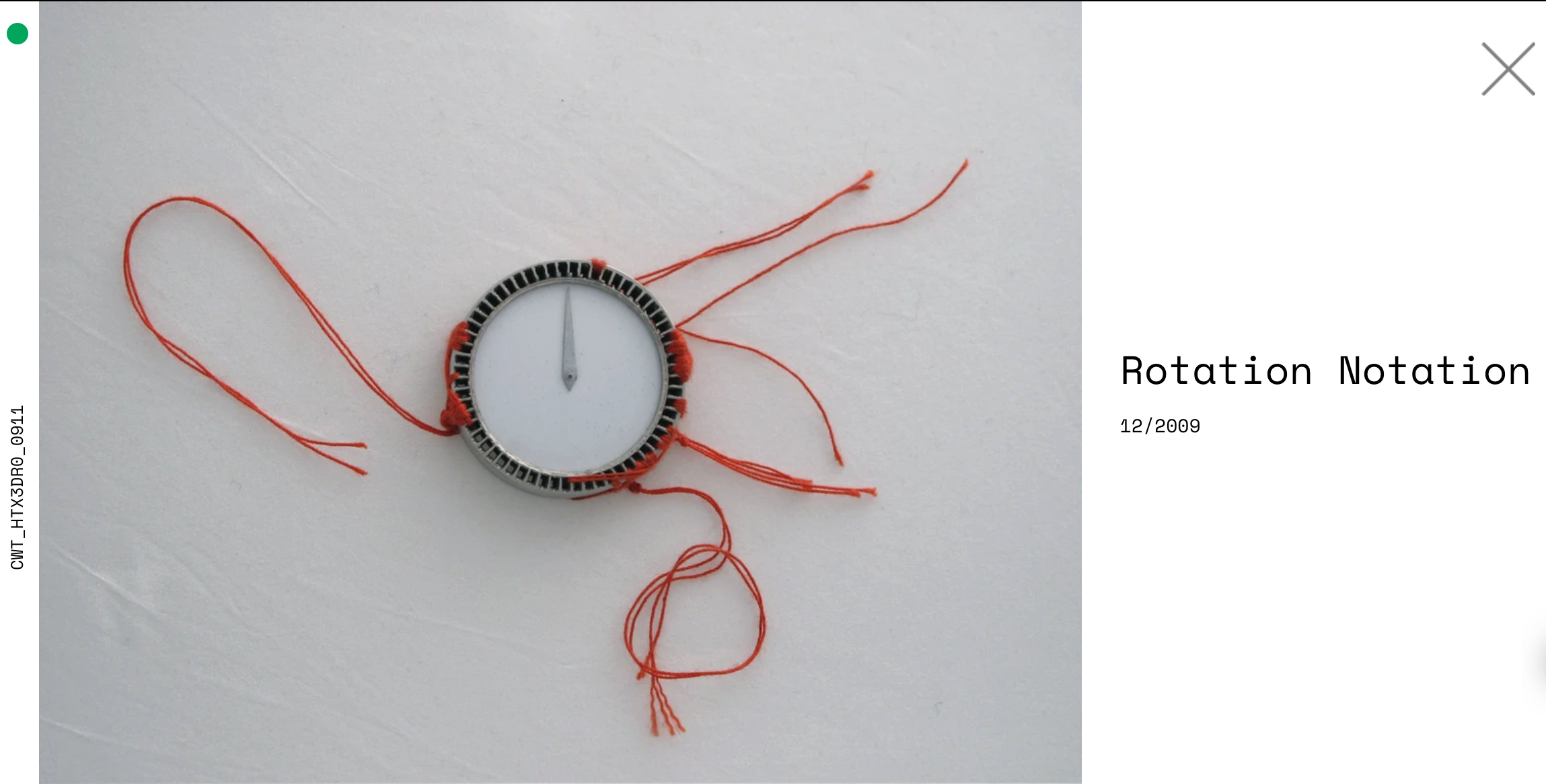
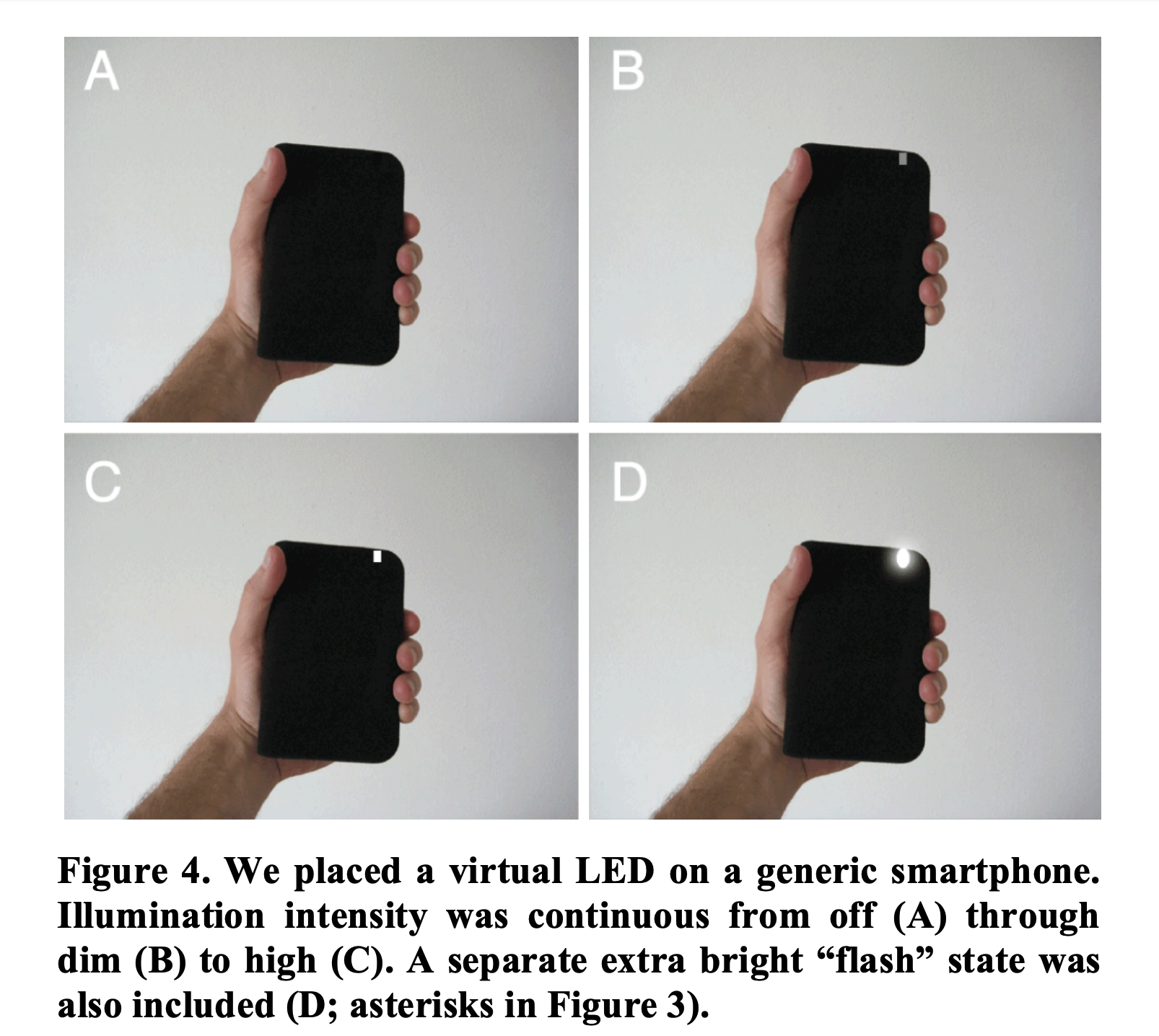
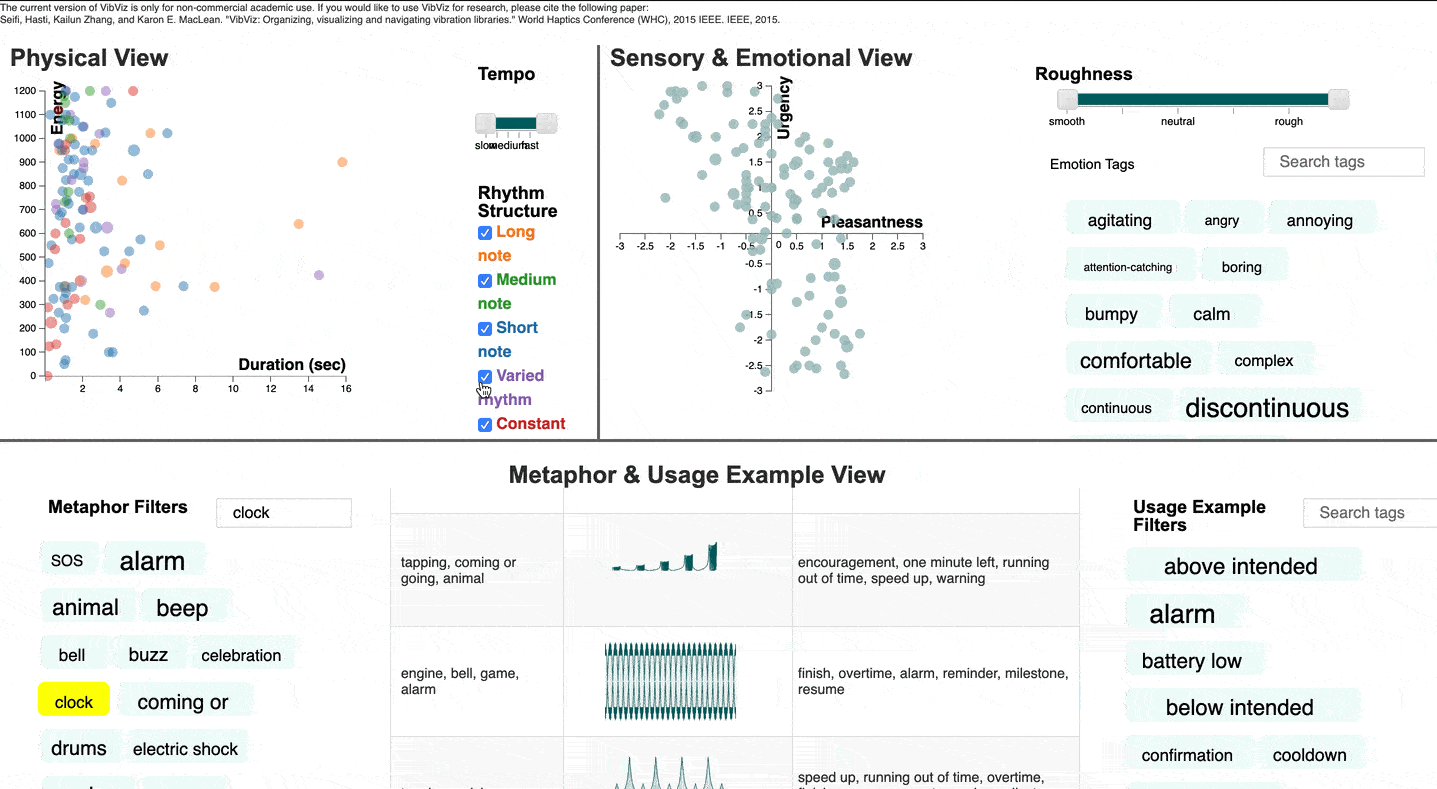
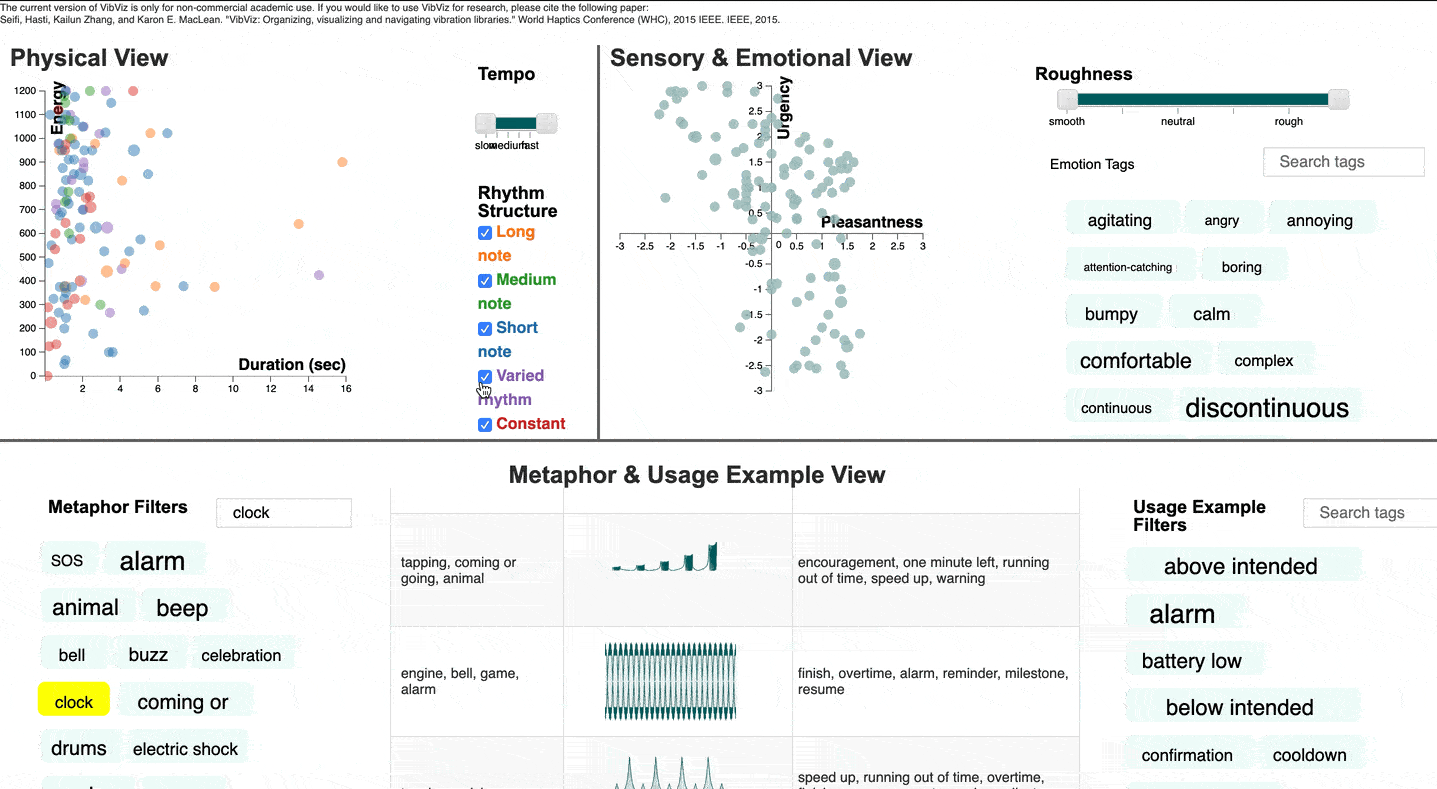
Inspiration
Professor Yao pointed us to work done by CW&T, specifically showing us how temporal information can be represented in non-standard forms. Professor Roberta Klatzky pointed us to the UBC SPIN Lab’s VibViz, an interactive analysis tool for choosing haptic patterns. Notable dimensions for these haptic patterns were “Urgency” and “Pleasantness,” which the team thought could be useful gauges when designing patterns for a novel haptic wearable device. Work by Professor Chris Harrison’s lab at CMU also pointed at creative ways to leverage stimuli as simple as a single LED. Prof. Harrison’s research on the expressive information available with just one LED gave us the motivation to pursue a haptic parallel to his light-based work.
Brainstorming
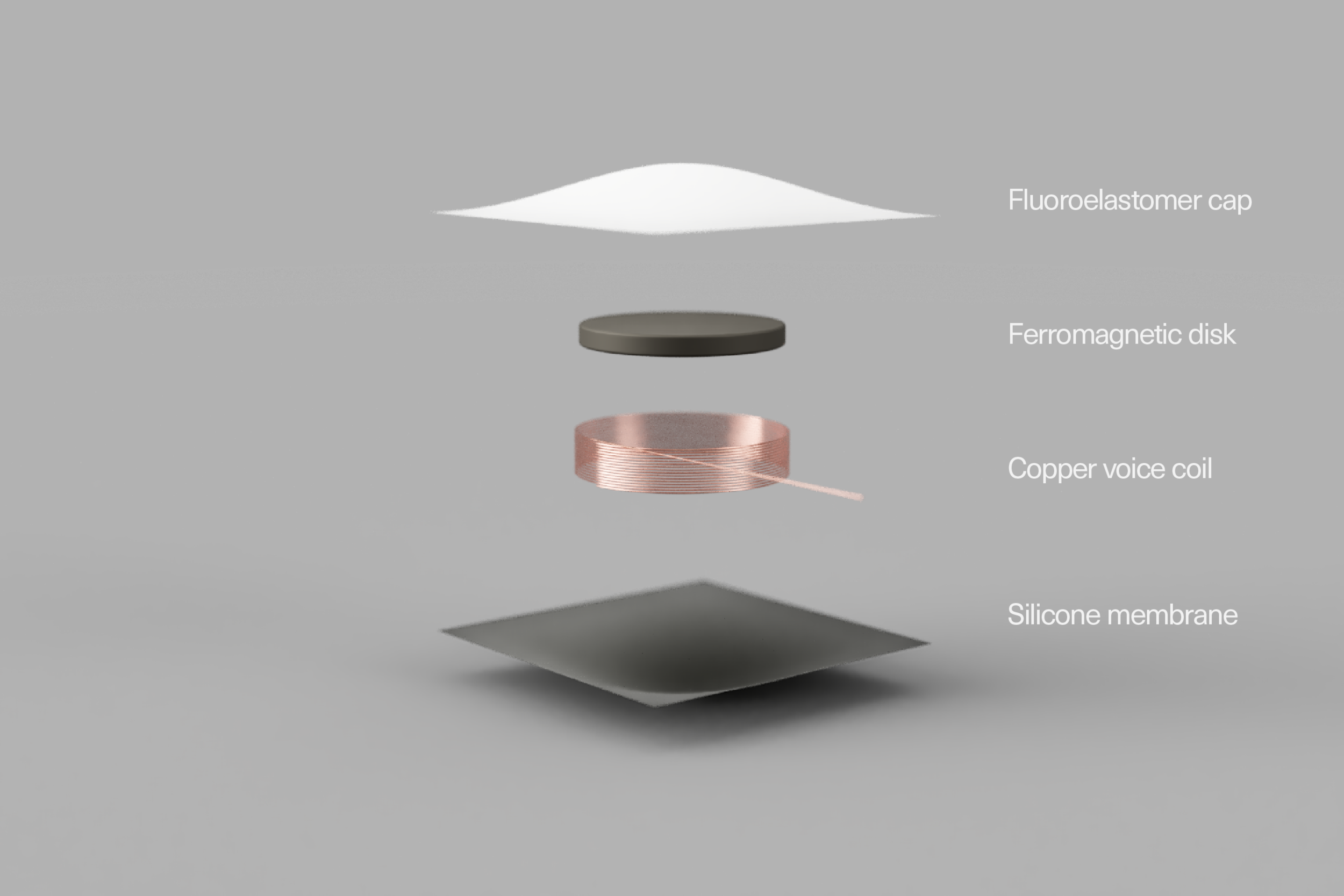
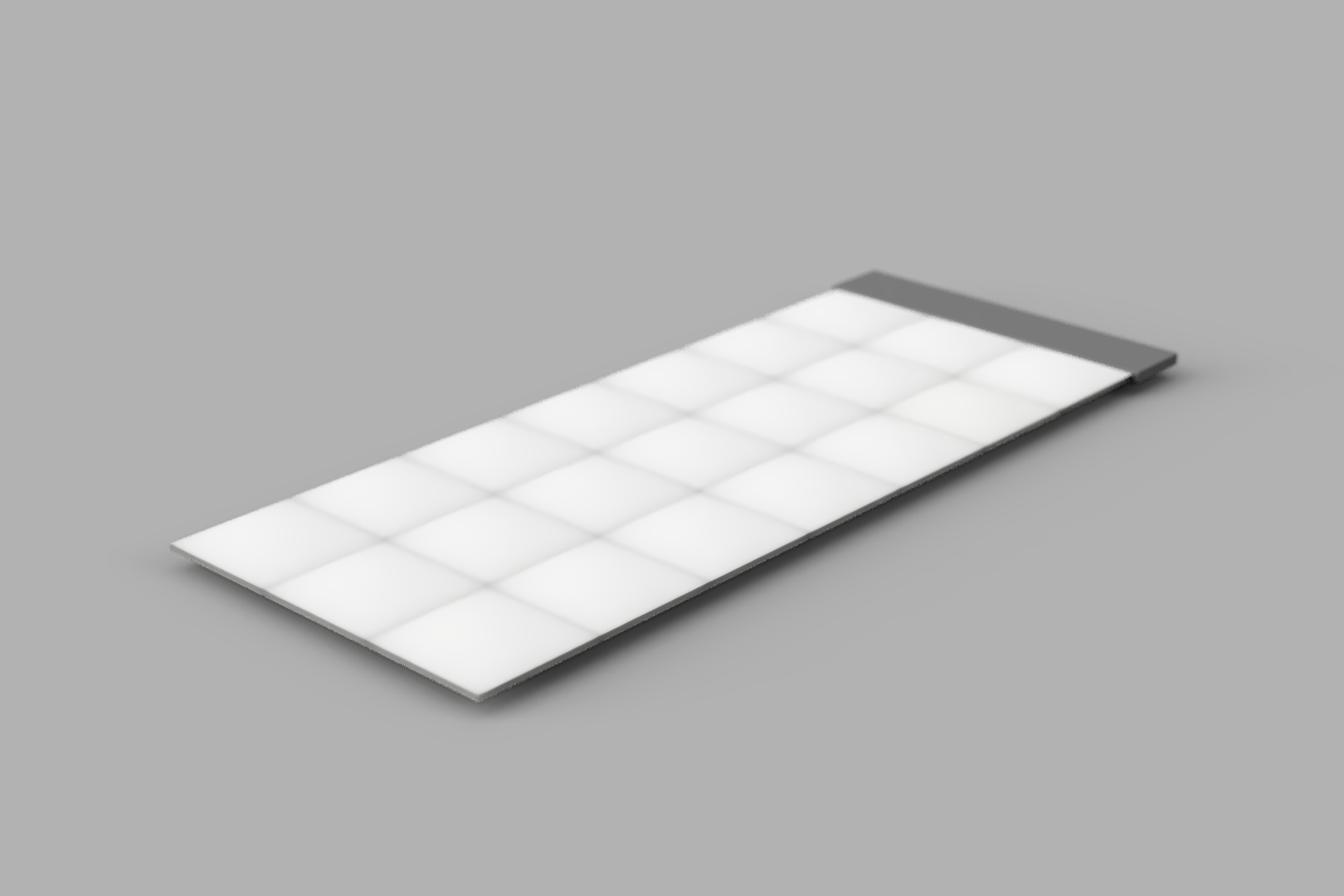
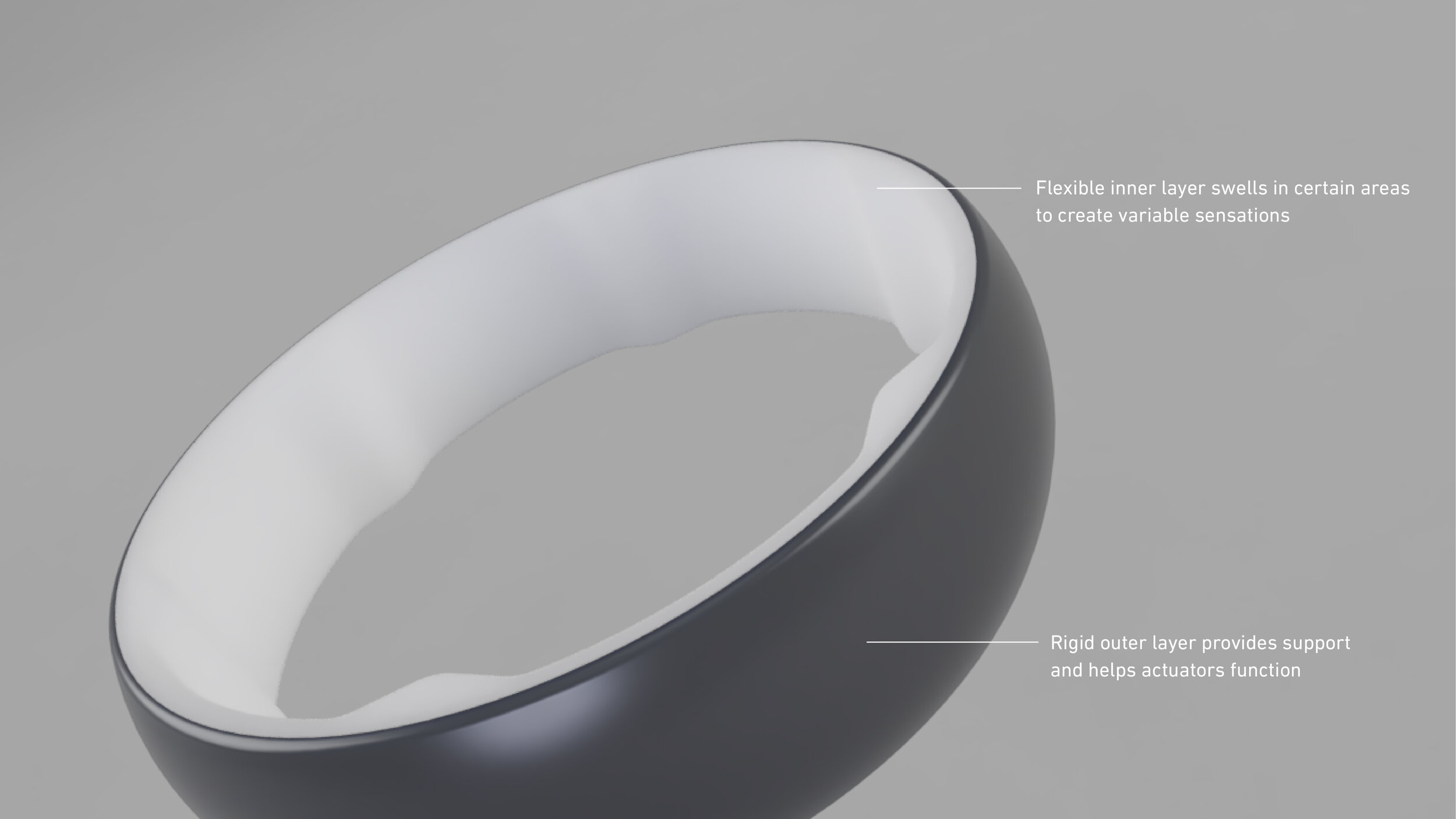
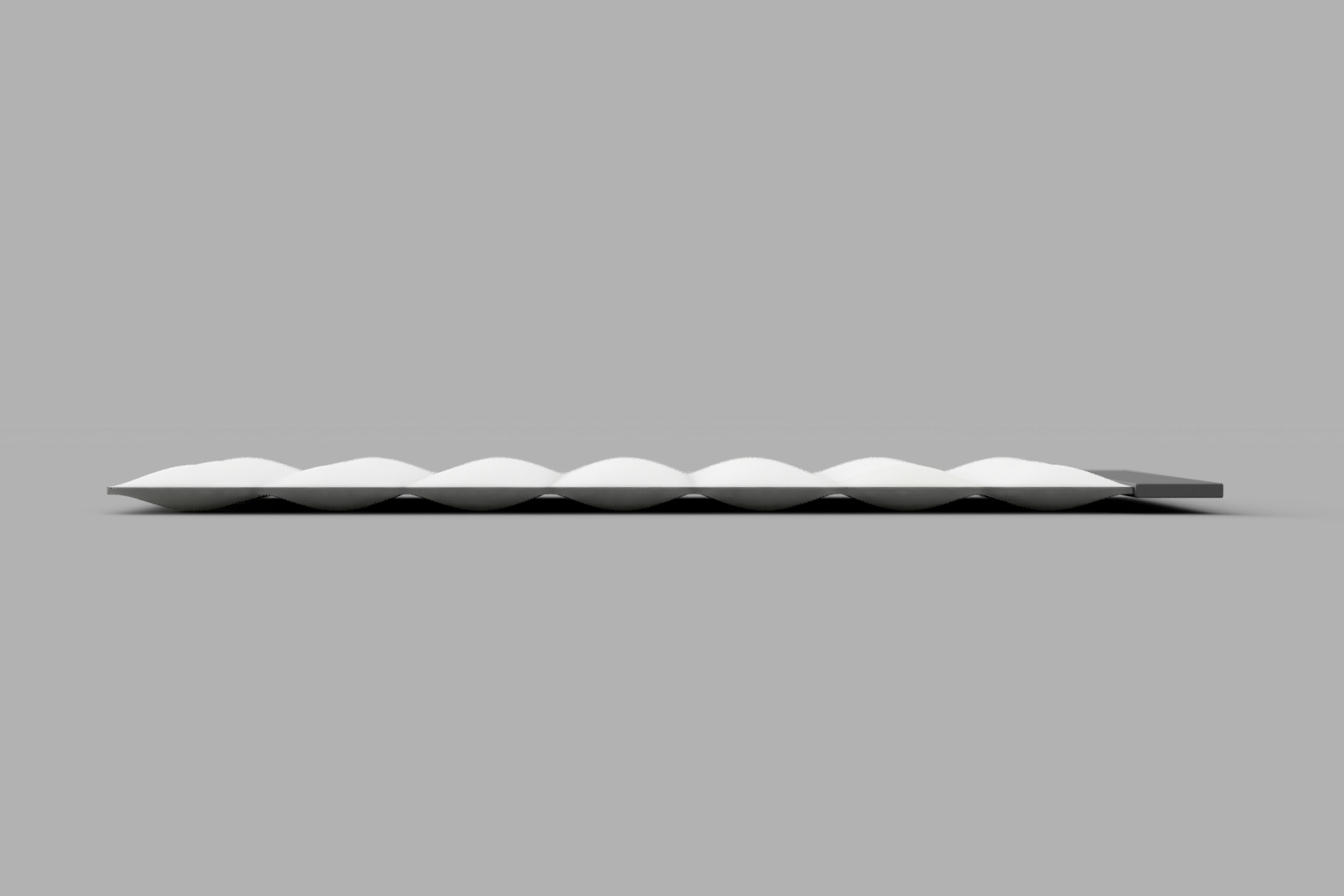
Ideation
The team loved the idea of a wrist-mounted haptic device. While the wrist is a very common choice for wearables, and we wanted to do something different, the wrist was the most sensible place to put a wearable. It was familiar, comfortable, and easily accessible to the user, so it made the most sense to design for this extremity. We white-boarded various mockups of this device, and settled on a version with 3 rows of 12 cells, enabling 36 possible simultaneous actuations. Each cell would essentially be a solenoid, composed of a copper wire shell and an inner magnetic core. This idealized prototype would give us a significant array of haptors to control, allowing us to create powerful and believable patterns. After agreeing on a design, Ian Shei and Eric Wong created 3D renderings of the model, to go out and gather further feedback from our peers and professor.
Prototype Construction and Testing
PROTOTYPING
After receiving positive feedback on our renderings and general concept, we created a low-fidelity version of this prototype, using only vibration motors. We created simple, easily differentiable patterns, and our user testers demonstrated the ability to distinguish each pattern. Anecdotally, our users demonstrated strong likability for these patterns, indicating that we were on the right track for further haptic pattern exploration.
Implementing Solenoids + Haptic Patterns
Solenoidal Prototype
We 3D printed a cylindrical enclosure for each cell, as well as a rigid PLA substrate to contain all 4 cells. Each cell had to be manually wound in enameled copper wire, and each magnet within the cell needed to be adhered to a PLA disc to allow the magnet to surpass the height of the cell enclosure. The white PLA substrate was flexible, and we eventually enclosed it with a clear plastic substrate. This would allow one side of the brace to remain still, while the other was pliable and moved with the solenoids.
Haptic Patterns
Simultaneously, we spent time creating animated Figma mockups of envisioned haptic patterns, so we could present them and receive feedback from users. While these patterns are for a 3x12 array of cells, we thought they could be implemented in a more abstracted form on our 1x4 cell prototype.
Final Prototype
Research Study
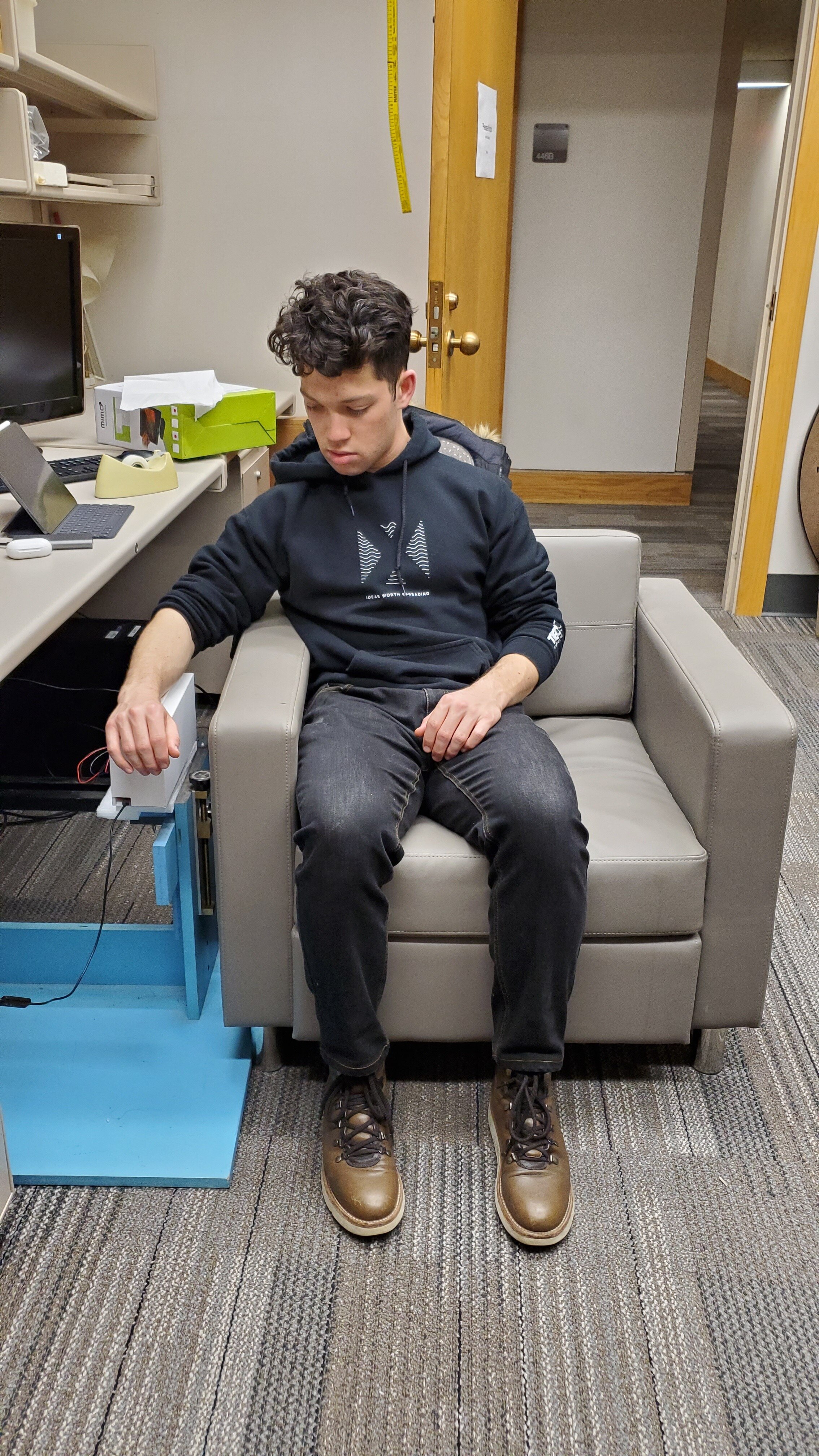
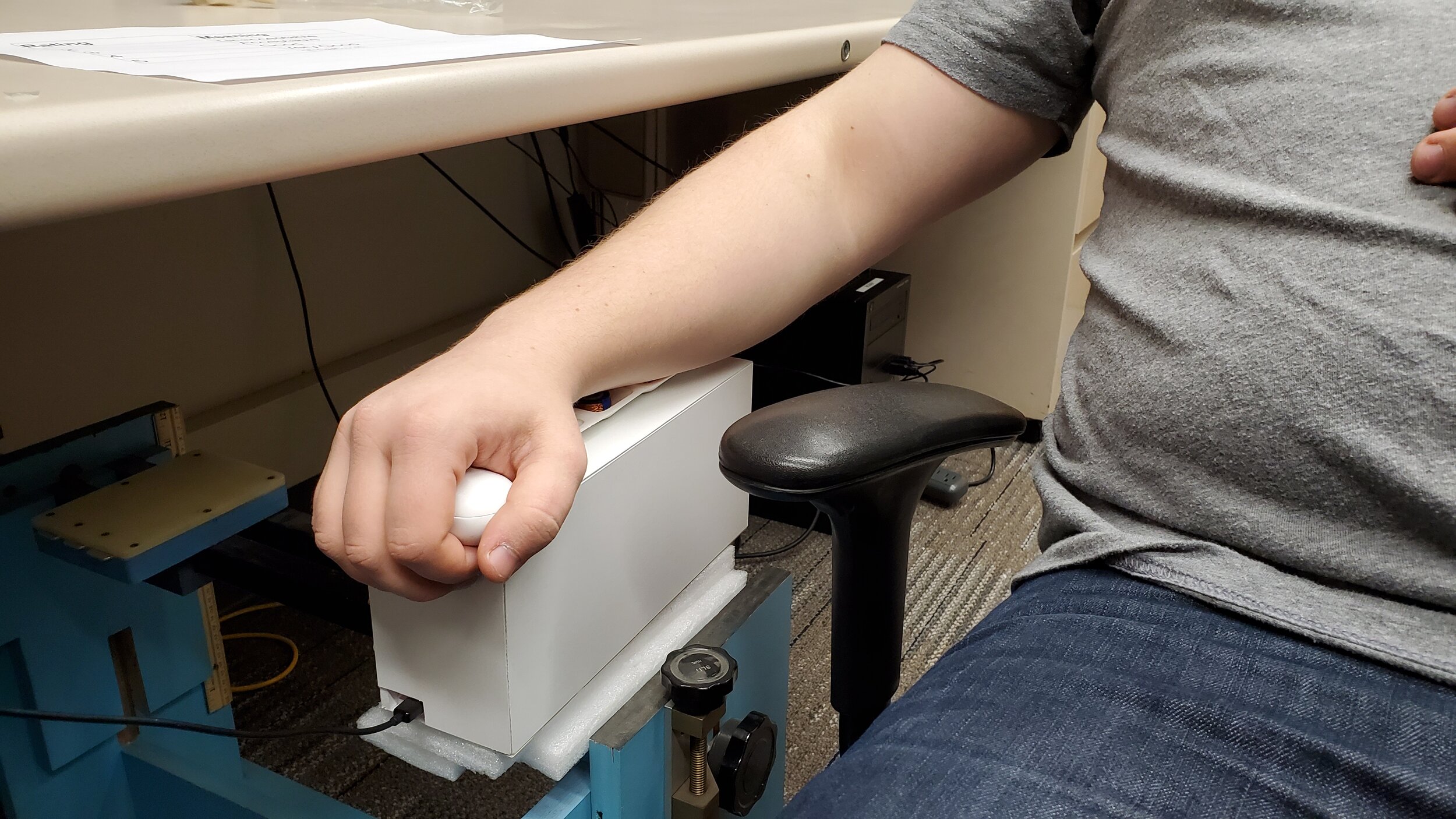
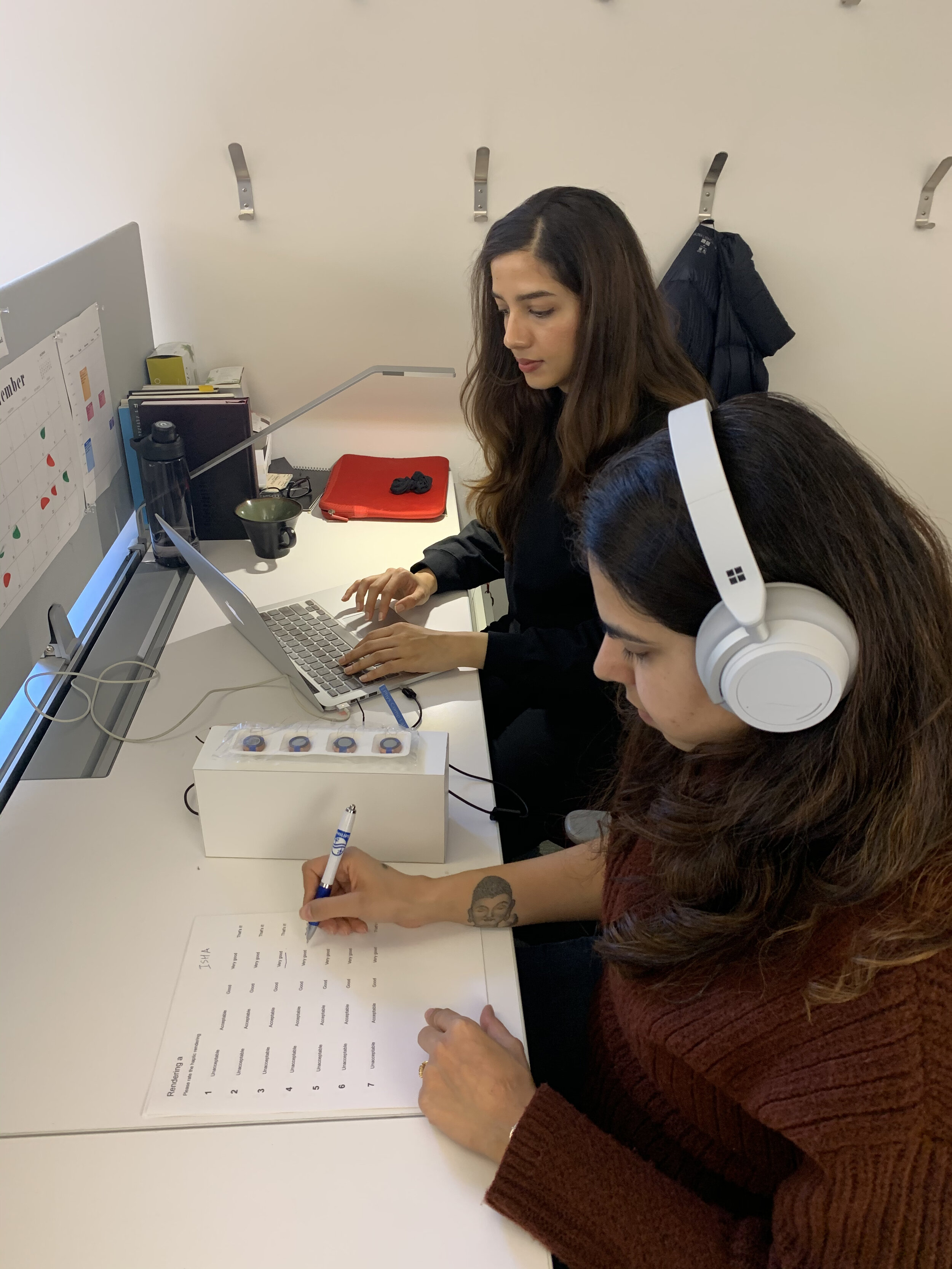
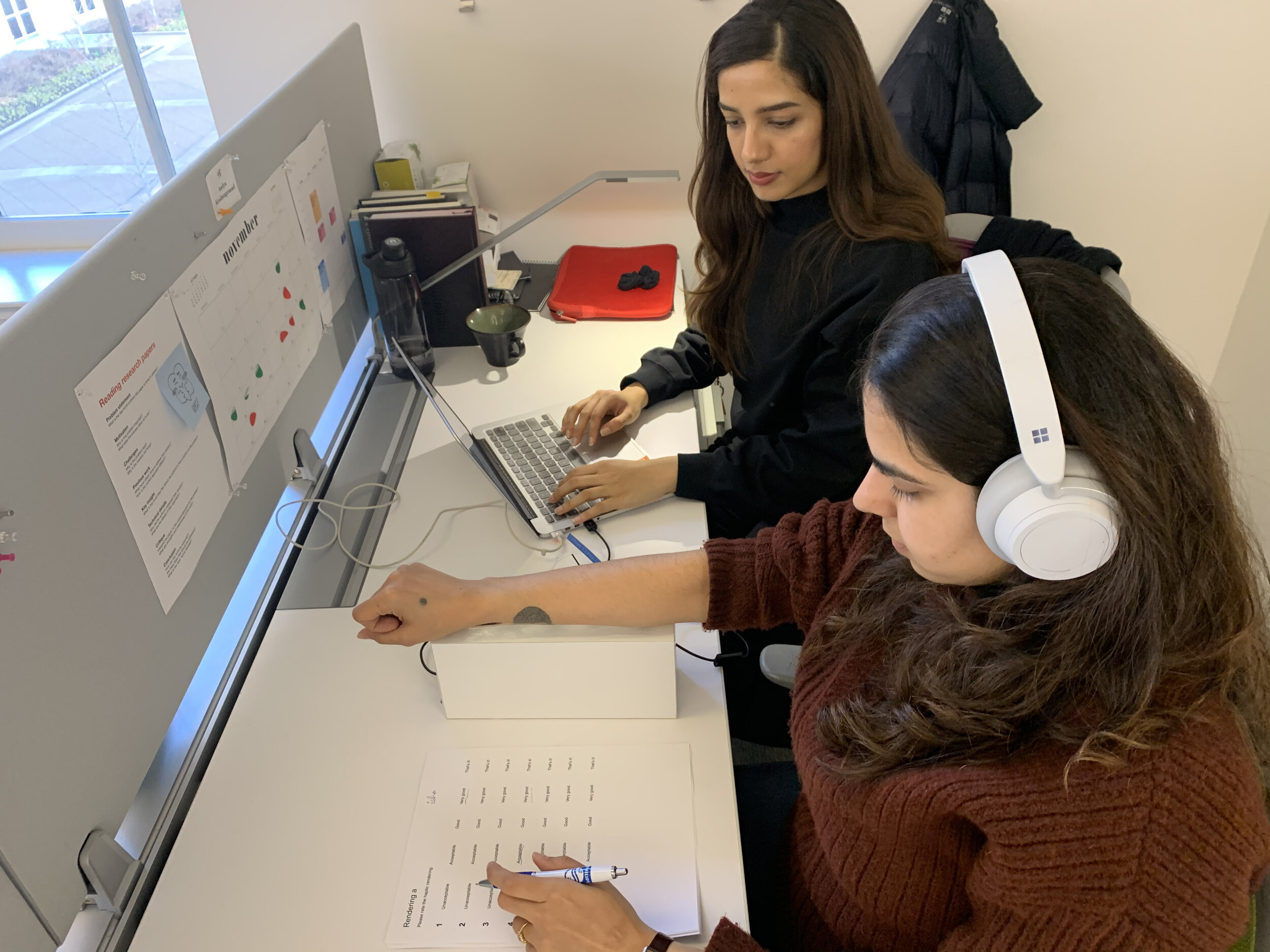
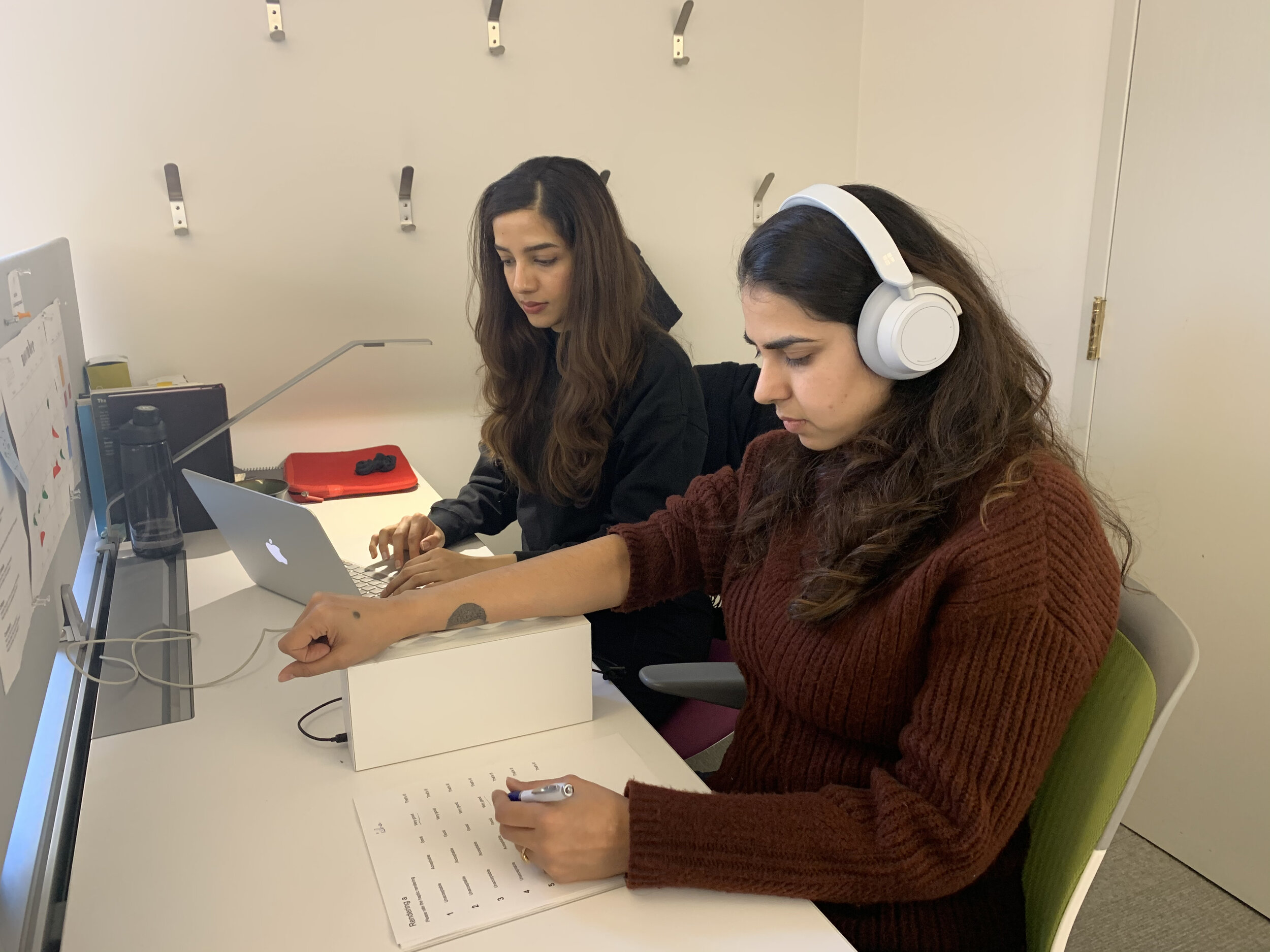
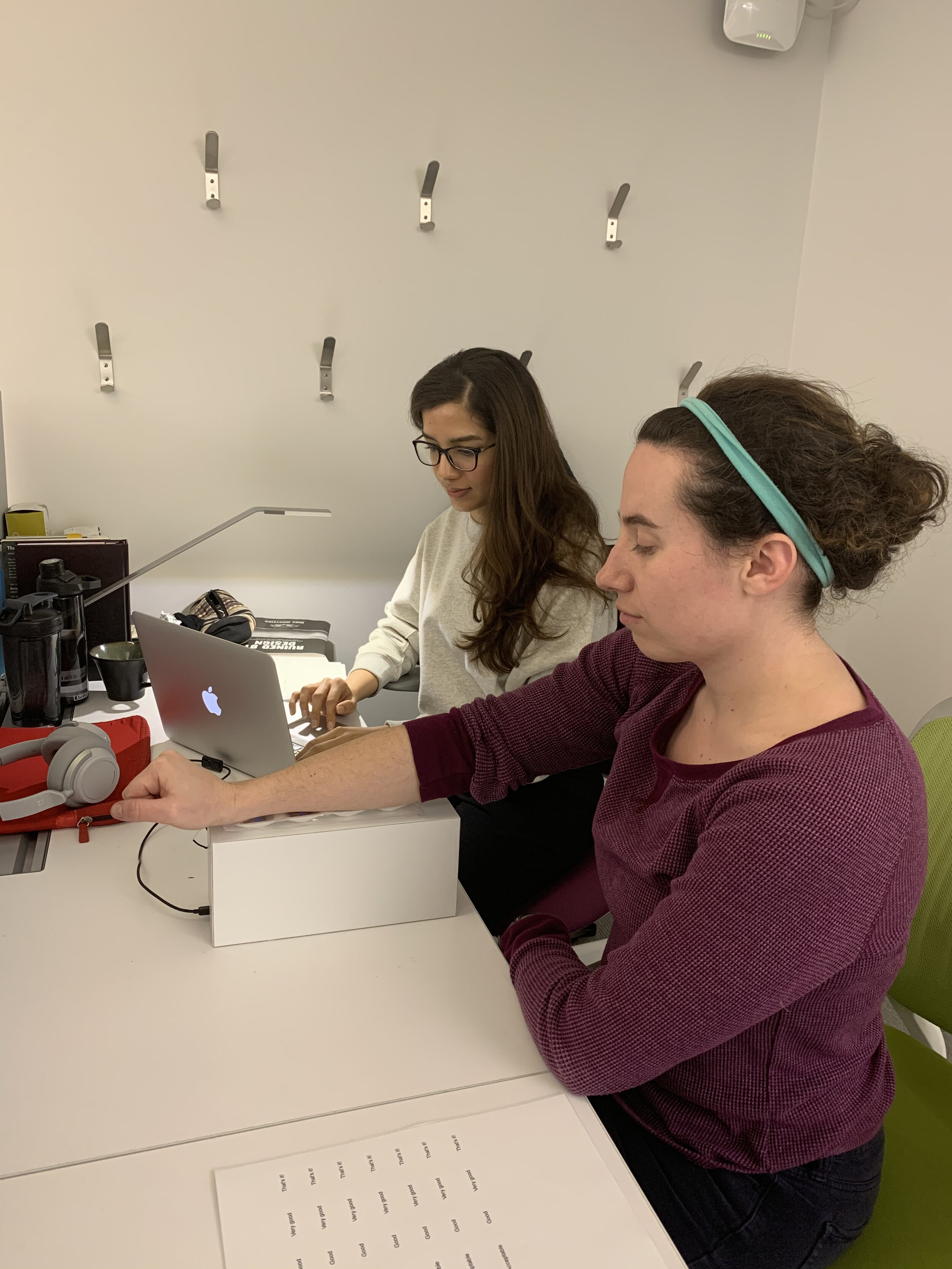
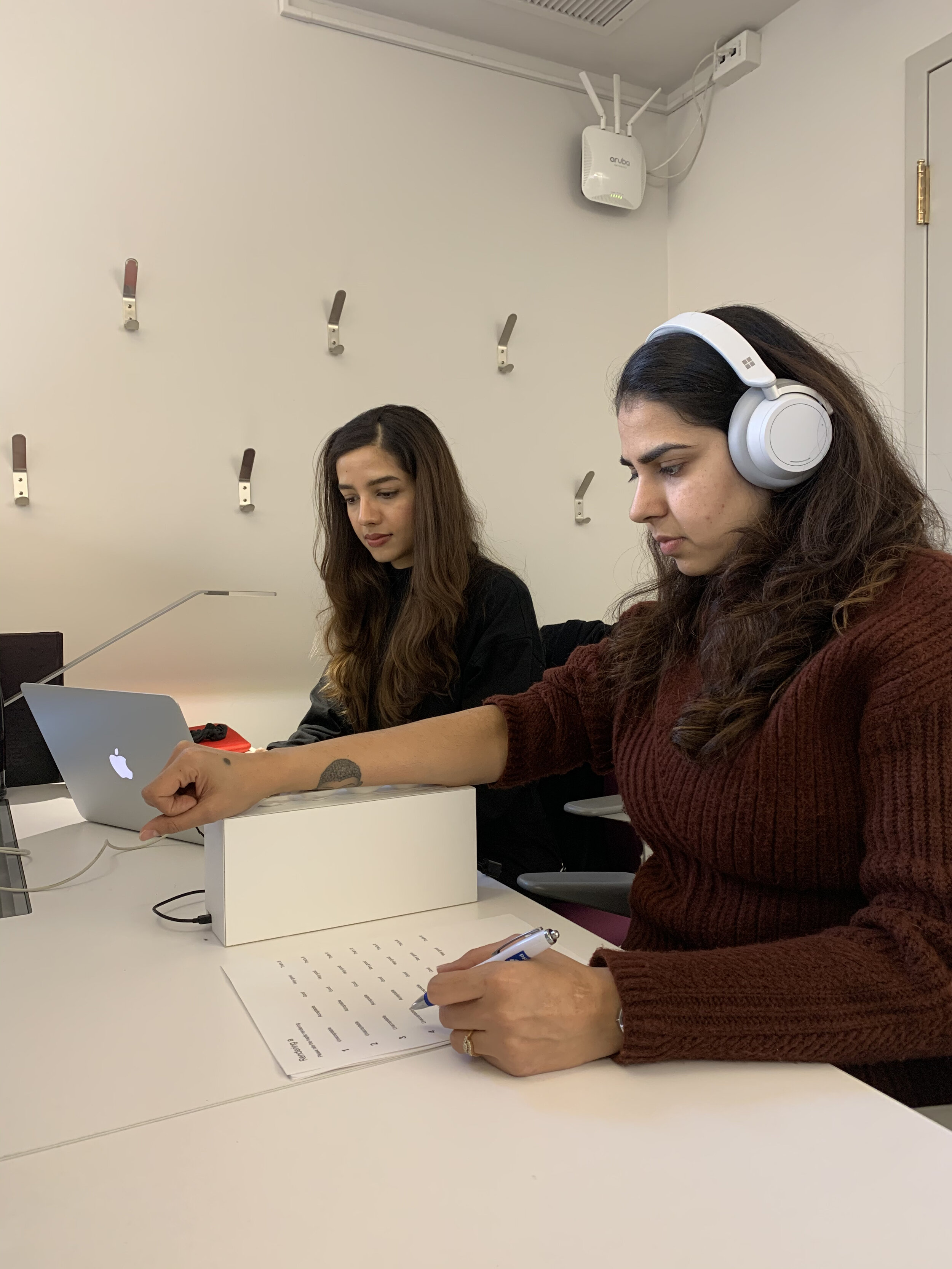
TESTING
We tested the final prototype in an unofficial study, and an official study. The unofficial study was run by Aadya Krishanaprasad, and asked participants to rate the effectiveness of a haptic pattern relative to a sound they were hearing through noise-cancelling headphones. Participants rated patterns like “calm heart beat,” “racing heart beat,” and “chopper,” on a 5 point Likert scale. The scale was: “Unacceptable” = 0, “Acceptable” = 1, “Good” = 2, “Very good” = 3, “That’s it” = 4. The mean rating for all of the patterns was above a 2, and the “Chopper” received a mean rating of 3.5, indicating strong acceptance.
The official study recruited 5 participants, 3 male, 2 female, mean age 20.4. The experiment tests young adults' rankings of haptic patterns and their corresponding real world counterparts. For example, “jumping tree frog” is a haptic pattern that attempts to simulate the feeling of a small frog jumping down a participant’s arm. There are 7 total patterns, with “Bad,” “Medium,” and “Good” variants of each pattern, for a total of 21 total haptic trials. Participants perform 42 total ranking trials, split into two parts, with a 5 minute break in-between. In Part 1, they are first given a practice round on a haptic pattern which is not used in any analysis, purely as a means to understand further tests, and the 5 point Likert ranking system they will use. After ranking the sample pattern, the participant will rank 21 randomly sorted haptic patterns from “Unacceptable,” “Acceptable,” “Good,” “Very good,” and “That’s it!”. After a five minute break, they will begin Trial 2. They will rank another 21 tests, using the same 21 samples from Trail 1, but in a randomized order.
Study Results
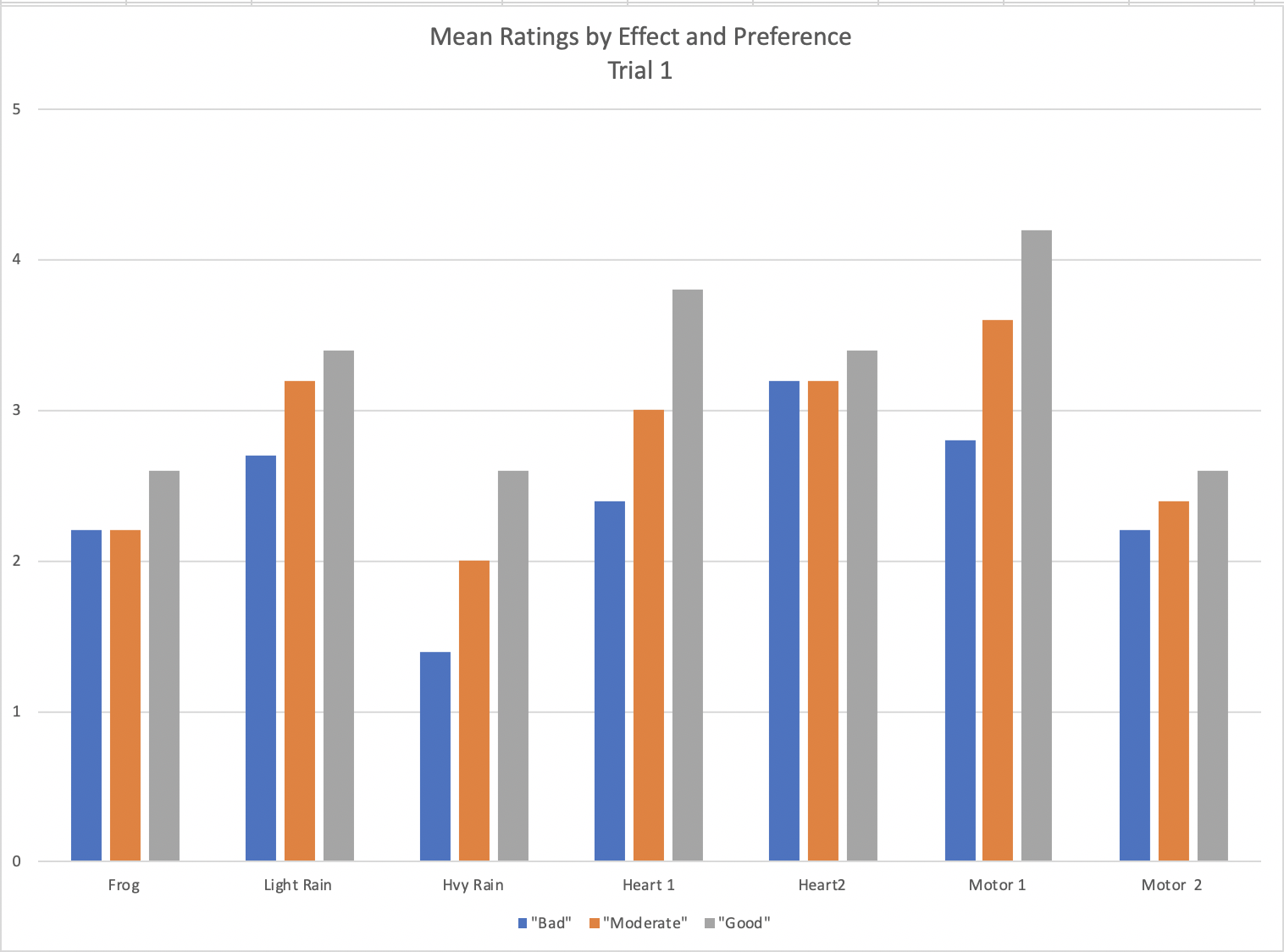
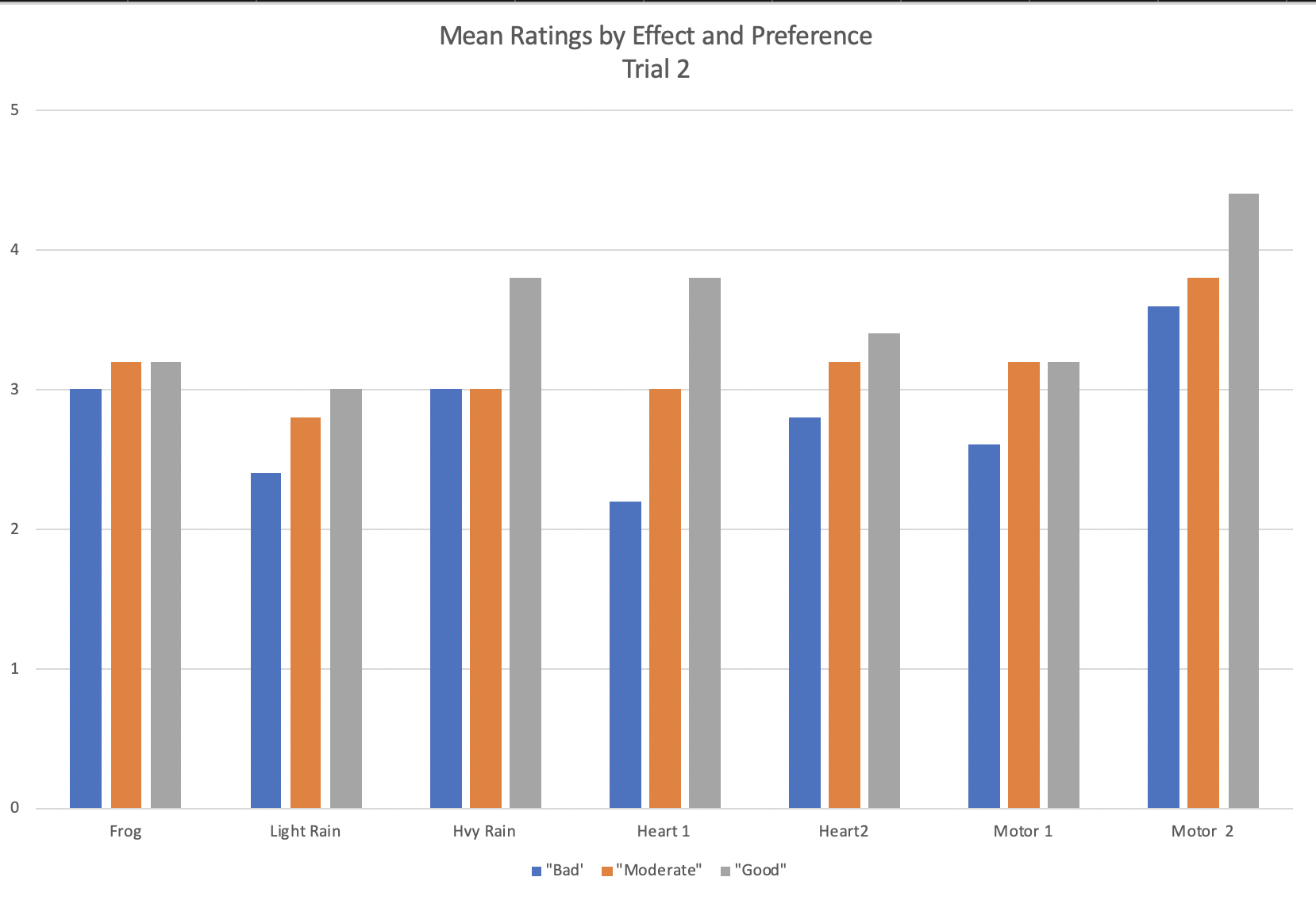
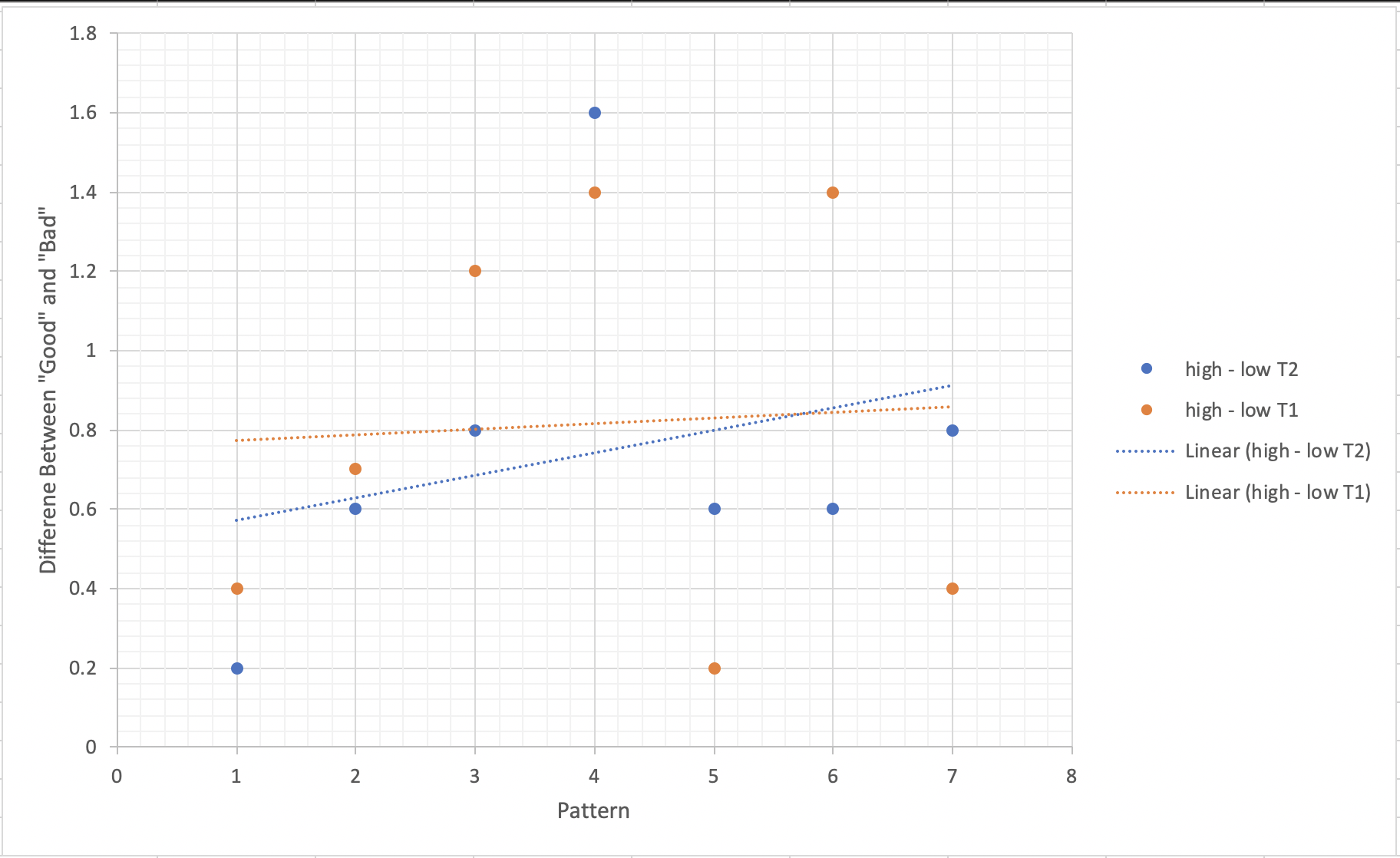
The results show that, across the board, the “good” (grey bar) variants of the patterns tended to have “good” scores of 3+, while the “bad” variants had ratings around and below the 2 range. Trial 1 showed lower overall acceptance than Trial 2, indicating that a bit of habituation could actually increase acceptance in most wearers. Additionally, as can be seen in the 3rd graphic above, the difference between scores for “Good” and “Bad” variants of each pattern decreased from Trial 1 to Trial 2 for most of the patterns in the study. This again indicates increasing acceptance of patterns after repeated exposure to stimuli. Additionally, the data shows a linear, strong, positive correlation between variant type and participant score. This implies that the patterns and variants used in this study were appropriately chosen in order of increasing believability, demonstrated by participant acceptance increasing from the “Bad” to “Good” variants across nearly all the patterns.